Serial Endosymbiosis Theory (SET)
“Much advance in evolution is due to the establishment of consortia between two organisms with entirely different genomes. Ecologists have barely begun to describe these interactions.” Ernst Mayr in foreword to Acquiring Genomes: A Theory of the Origins of Species, by Lynn Margulis and Dorion Sagan.
In the 1960’s, biologist Lynn Margulis actively promoted endosymbiotic theory – The Endosymbiotic Theory of Eukaryote Evolution. Margulis published "Symbiosis in Cell Evolution" in 1981. Even though the essential idea had a lengthy history, mainstream biologists initially reacted to Margulis’ claims with incredulity and ridicule. On the basis of experimental evidence, Serial Endosymbiotic Theory (SET) is now almost universally accepted as the most plausible explanation for evolution of eukaryotes.
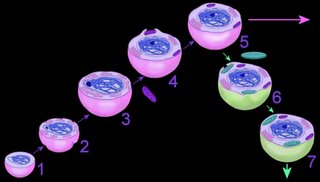
Ultimately, both the prokaryotic host and the bacteria endosymbionts developed an interdependence through which both entities lost their ability to function without the other (5). It is assumed that Cyanobacteria-generated oxygen in the early atmosphere necessitated endosymbiotic metabolic association between ingested aerobic bacteria and anaerobic host prokaryotes. The ingested bacteria ultimately performed oxidative metabolism necessary to the survival of the original host cell, which would otherwise have been poisoned by atmospheric oxygen. The former free-living aerobic bacteria assumed the role of mitochondria within its host cell (purple organelles within 5).
Similarly, serial ingestion of photosynthetic bacteria by endosymbiontic prokaryotes or eukaryotes (5-6) led to the evolution the ancestors of eukaryotic plants and photosynthetic protists (7). As the ingested photosynthetic bacteria adapted to the ingesting prokaryotic host cell, plastids, such as the chloroplast evolved (green organelle within 7). Primary plastids are found in Chlorophyta and plants, Rhodophyta, and Glaucocystophyta because their plastids are derived directly from a Cyanobacterium. All other lineages of plastids have arisen through secondary (or tertiary) endosymbiosis, in which a eukaryote already possessing plastids is engulfed by a second eukaryote. Considerable gene transfer has occurred among genomes and, at times, between organisms. A particularly complex history of plastid acquisition is found in eukaryotic crown group Alveolata. diagrams
Prior to Margulis' conception of the Symbiotic Theory in the 1960's, biologists believed that the eukaryote's nuclear DNA coded for cellular organelles. When Margulis initially proposed the Symbiotic Theory, she predicted that organelles of prokaryotic origin would be coded for by their own DNA. In the 1980's, evidence in support of Margulis’ prediction was found in the distinct prokaryotic-DNA of the mitochondria and chloroplasts of eukaryotic cells.